Pretty well everyone with a passing interest in cancer will know that it’s complicated and that a lot of that complexity arises because the mass of cells we call a tumour is a real mixture. Scores of different types of cell coming and going in an ever-changing dynamic structure. By and large primary tumours can be dealt with by surgery or radiotherapy but the big problem is that some tumour cells can change their state over time and become metastatic — i.e. they can leave the primary site, migrate and found a new tumour colony elsewhere in the body. Currently rather little is known about how this happens but what we do know is that most cancer deaths are caused by these ‘secondaries’ because they are extremely difficult to treat.
Tracking the culprit
So a big question in cancer biology is: what gives cells the power to metastasize? In a technical tour de force Dian Yang, Jonathan Weissman and colleagues at the Whitehead Institute for Biomedical Research in Cambridge, Massachusetts set out to chart a tumour’s journey from its origins as a single cell to metastasis.
The basic idea
They genetically engineered mice to track the accumulation of mutations in their cells. After activating two powerful ‘driver’ mutations in lung cells, tumours were allowed to grow for up to six months before harvesting each tumour and examining individual cells.
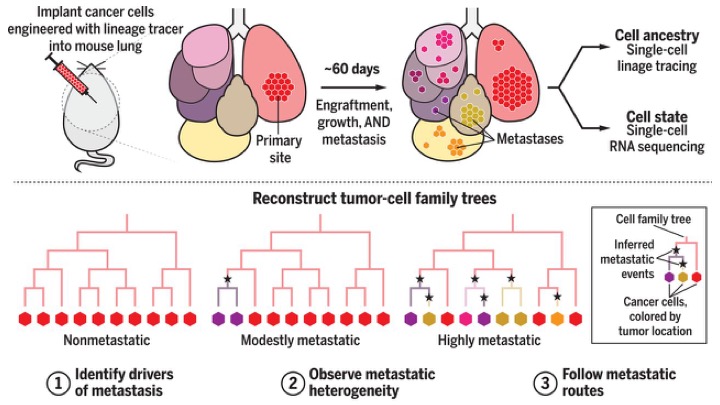
Following cancer through the body at the single cell level. The method combines CRISPR-Cas9 genome editing and single-cell RNA-sequencing. Editing introduces barcodes into cells permitting individual cells to be followed. Engineered metastatic human cells are implanted in the lungs of mice (a mouse xenograft model). After harvesting cell ancestry can be traced. Subclones have different gene expression profiles that associate with being non-metastatic, modestly metastatic or highly metastatic. From Quinn et al. 2021.
The result was an assembly of family trees of cancer cells in the tumour. Each subpopulation of cells had a unique gene-expression pattern. Some of these patterns conferred greater capacity for multiplying and surviving – and those subpopulations eventually dominated the tumour and allowed it to metastasize.
For molecular nerds: a bit more technology
The scheme above sketches the general idea but the methods used are such a stunning demonstration of what technology can now do it’s worth explaining some of the detail. The starting point was mouse embryonic stem cells (mESCs) engineered to have to extra bits so that two ‘drivers’ (a Kras oncogene and knock-out of the P53 tumour suppressor gene) can be turned on, when required, to start lung tumour development.
In addition CRISPR/Cas9 generated double-stranded breaks that result in heritable insertions or deletions (indels). This was achieved using a single piggyBac transposon vector (this is a movable genetic element that efficiently shifts between vectors and chromosomes through a “cut-and-paste” mechanism). The sperm injected into the eggs (oocytes) carried Rosa26::Cas9:EGFP (ROSA26 is a locus that gives continuous expression of genes in mice; EGFP = enhanced green fluorescent protein so that all cells can be seen by fluorescence and hence tracked after fertilization). For this to work they also injected transposase mRNA.
Injection of a transposon vector, a transposase mRNA and Rosa26::Cas9:eGFP sperm into oocytes. This ensures tracing in all subsequent cells. From Chan et al. 2019.
Clones of mESCs were selected and injected into blastocysts (a cluster of dividing cells made by a fertilized egg). This produces KP-Tracer chimeric mice (their tissues being composed of cells from both the genetically modified mESCs and the genetically wild-type host blastocyst, as evidenced by the patched coat colours of these mice).
Generation of the KP-Tracer chimeric mice and initiation of KP-Tracer tumours. After six months individual tumours are dissociated into single-cell suspensions and single-cell sequencing libraries are prepared. From Yang et al. 2022.
Subsequent administration via the trachea of a barcoded lentivirus expressing Cre recombinase switches on the drivers and tumour development.
What did we learn?
Most surprisingly, tumours that evolve from one individual cancer cell have big differences in their capacity to spread to distant sites (metastasize). Some are non-metastatic — they never leave the primary tumour. Others become highly aggressive and frequently shift their location. Remarkably, the range of metastatic cells that arises in these mice stems from pre-existing, heritable cellular states. In other words, the heterogeneity in the expression of metastasis-associated genes existed in the mESCs before they were implanted in mice. Those with the most potent metastatic transcriptional signatures went on to be more metastatic in vivo. Cells from the same clone implanted into different mice show almost identical metastatic behaviour — an important point in validating the model.
Not surprising was that single-cell RNA sequencing revealed active genes already known to be associated with metastasis in lung cancers. However, novel culprits were also identified including the up-regulation of Cd24a and interleukin 33 (Il33). Il33 is considered to be an ‘alarmin’ that modulates the interaction between cancer cells and the immune system promoting tumour development.
Stunning stuff but what next?
An encouraging finding was that components of the signatures of aggressive tumours found in the mouse model have also been shown to be predictive of human disease outcome. Which, of course, implies that if the genetic changes leading to metastasis can be identified early in human cancers, targeting those subpopulations might be a step towards blocking the most critical step in cancer progression.
References
Quinn JJ, Jones MG, Okimoto RA, Nanjo S, Chan MM, Yosef N, Bivona TG, Weissman JS. Single-cell lineages reveal the rates, routes, and drivers of metastasis in cancer xenografts. Science. 2021 Feb 26;371(6532):eabc1944. doi: 10.1126/science.abc1944. Epub 2021 Jan 21. PMID: 33479121; PMCID: PMC7983364.
Chan MM, Smith ZD, Grosswendt S, Kretzmer H, Norman TM, Adamson B, Jost M, Quinn JJ, Yang D, Jones MG, Khodaverdian A, Yosef N, Meissner A, Weissman JS. Molecular recording of mammalian embryogenesis. Nature. 2019 Jun;570(7759):77-82. doi: 10.1038/s41586-019-1184-5. Epub 2019 May 13. PMID: 31086336; PMCID: PMC7229772.
Yang D, Jones MG, Naranjo S, Rideout WM 3rd, Min KHJ, Ho R, Wu W, Replogle JM, Page JL, Quinn JJ, Horns F, Qiu X, Chen MZ, Freed-Pastor WA, McGinnis CS, Patterson DM, Gartner ZJ, Chow ED, Bivona TG, Chan MM, Yosef N, Jacks T, Weissman JS. Lineage tracing reveals the phylodynamics, plasticity, and paths of tumor evolution. Cell. 2022 May 26;185(11):1905-1923.e25. doi: 10.1016/j.cell.2022.04.015. Epub 2022 May 5. PMID: 35523183.
Pingback: Channeling Metastasis | Betrayed by Nature: The War on Cancer